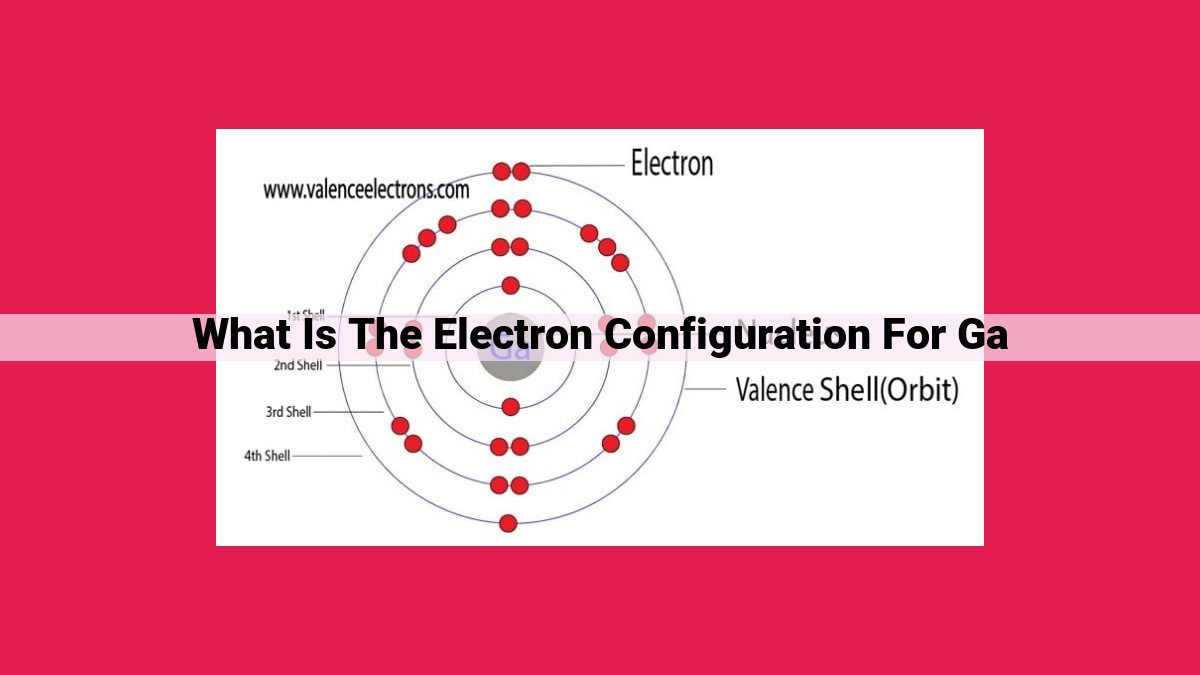
Gallium’s Electron Configuration: Unlocking Reactivity And Behavior
Electron Configuration for Gallium: Gallium, an element with atomic number 31, exhibits an electron configuration of 1s22s22p63s23p63d104s24p1. This configuration reveals that gallium possesses two valence electrons in its outermost 4p orbital. Valence electrons play a crucial role in chemical bonding, determining an element's reactivity and behavior.
Atomic Number and Mass Number: Unraveling the Heart of Atoms
Every atom, the fundamental building block of the universe, holds a unique identity defined by two crucial numbers: atomic number and mass number. Understanding these numbers is like unlocking the secret code that grants us intimate knowledge of an atom's structure and behavior.
Atomic Number: The Fingerprint of an Element
The atomic number is akin to an atom's unique fingerprint, a number that identifies the element it belongs to. It represents the number of protons, positively charged particles that reside in the atom's nucleus. The atomic number is an immutable characteristic, dictating an element's position on the periodic table and its chemical properties. For instance, hydrogen, the simplest element, has an atomic number of 1, indicating that it possesses a single proton in its nucleus.
Mass Number: A Measure of Atomic Mass
The mass number provides a glimpse into an atom's mass. It is the sum of the number of protons and neutrons, particles found alongside protons in the nucleus. Neutrons, while electrically neutral, contribute to an atom's overall mass. The mass number provides valuable information about the relative heaviness of different atoms. For example, carbon-12, a commonly used isotope of carbon, has a mass number of 12, indicating that it contains six protons (atomic number 6) and six neutrons in its nucleus.
By unraveling the mysteries of atomic number and mass number, we gain a deeper understanding of the atomic realm, laying the groundwork for exploring the intricate world of chemistry and its transformative applications.
Valence Electrons: The Key Players in Chemical Reactions
In the realm of chemistry, there's a group of electrons that take center stage: valence electrons. Picture them as the extroverted members of the electron family, eagerly participating in chemical reactions and shaping the behavior of molecules.
What's Their Role?
- Chemical Reactions: Valence electrons are the ultimate matchmakers in chemistry. They're the ones that jump between atoms, forming ionic and covalent bonds to create new molecules.
- Electronegativity: This term measures how strongly an atom attracts valence electrons from other atoms. The higher the electronegativity, the more greedy an atom is for electrons.
- Behavior: The number of valence electrons determines an atom's chemical properties. For example, elements with a complete valence shell (eight electrons) are highly stable and unreactive, while those with only a few valence electrons are more reactive.
Dive into the World of Gallium
Let's take gallium, an element in Group 13 of the periodic table, as an example. Gallium has three valence electrons, making it a relatively reactive metal. These valence electrons play a crucial role in its chemical reactions and physical characteristics.
Building Electron Configurations: The Rules of Engagement
The electron configuration of an atom describes the arrangement of its electrons in orbitals. To build it, we follow two fundamental principles:
- Aufbau Principle: Electrons fill orbitals in order of increasing energy, from lowest to highest.
- Hund's Rule: Electrons within a subshell prefer to have parallel spins (aligned in the same direction).
The Periodic Puzzle
The periodic table is a roadmap of elements, arranged according to their atomic numbers and electron configurations. Elements in the same group (vertical column) share similar electron configurations and properties. For instance, gallium is part of Group 13, along with aluminum and indium, all of which have three valence electrons.
Section 1: Electron Configuration: Mapping Atomic Electrons
Picture electrons as tiny dancers, each with a unique energy level and spin. In every atom, these dancers occupy specific orbitals, regions of space where they're most likely to be found. To map this electron choreography, scientists use a set of rules and principles.
Quantum mechanics, a branch of physics, tells us that not all orbitals are created equal. Each is characterized by quantum numbers, like energy levels, shapes, and spins. These numbers describe the specific properties of each electron.
Orbitals, like the stages in a theater, come in different shapes and sizes. They're named with letters (s, p, d, f) and numbers. The s orbitals are spherical, like little clouds surrounding the nucleus. p orbitals are dumbbell-shaped, with two lobes pointing in opposite directions.
Electrons occupy these orbitals in a particular order, following the Aufbau principle. They fill the lowest energy orbitals first, like dancers taking their places on the lowest steps of a stage. Only when the lower orbitals are filled can electrons move to higher energy ones.
Hund's rule adds another twist to the dance. It says that when multiple electrons occupy the same orbital, they prefer to have their spins aligned. This is like dancers spinning in unison, creating a coordinated performance.
Mapping electron configuration is like deciphering a secret code. It reveals the inner workings of atoms, their chemical behavior, and their place in the periodic table.
Orbital Notation: Unraveling the Architecture of Electrons
In the realm of atomic structure, we encounter the fascinating concept of electron configuration, which describes the meticulous arrangement of electrons within the atom's orbitals. Each electron resides in a specific orbital, characterized by its unique energy level and shape.
To decipher this intricate arrangement, we resort to the power of quantum numbers. These are numerical descriptors that define the properties of each orbital.
- Principal Quantum Number (n): Indicates the energy level of the orbital, with higher numbers denoting higher energy levels.
- Azimuthal Quantum Number (l): Specifies the shape of the orbital, corresponding to different sublevels denoted by letters (s, p, d, f).
- Magnetic Quantum Number (ml): Describes the orientation of the orbital in space within a sublevel.
- Spin Quantum Number (ms): Indicates the direction of the electron's spin, either clockwise (+) or counterclockwise (-).
Using these quantum numbers, we can determine the exact location and properties of each electron within an atom. By combining the values of the quantum numbers, we obtain a unique orbital notation that serves as a blueprint for the atom's electron configuration.
For instance, the notation "3p" represents an electron occupying a p orbital in the third energy level. The subscript "x" indicates further that the orbital is oriented along the x-axis in space. This notation provides a concise and informative description of the electron's position and properties within the atom.
Gallium's Electron Configuration: Analyze the arrangement of electrons in gallium's orbitals, considering subshells and energy levels.
Understanding Gallium's Electron Configuration
In the realm of chemistry, understanding the electron configuration of elements holds paramount importance for unraveling their chemical behavior and properties. Among these elements, gallium stands out for its intriguing electron arrangement.
Gallium, a silvery-white metal, occupies the 13th position on the periodic table, sharing the group with elements like aluminum, indium, and thallium. Its electron configuration, the distribution of its electrons in orbitals around the atomic nucleus, plays a pivotal role in shaping its unique characteristics.
To decipher gallium's electron configuration, we embark on a journey through the intricate world of atomic structure. Each orbital, like a celestial sphere, represents a region of space where an electron is most likely to reside. These orbitals are characterized by three quantum numbers: the principal quantum number (n), which determines the energy level; the angular momentum quantum number (l), which describes the shape of the orbital; and the magnetic quantum number (ml), which specifies its orientation in space.
Gallium's electron configuration can be systematically constructed by adhering to the guiding principles of quantum mechanics. Starting with the lowest energy level (n = 1), we fill the 1s orbital with two electrons. As we move to the next energy level (n = 2), we encounter the 2s and 2p orbitals. The 2s orbital accommodates two electrons, while the 2p orbitals can hold a total of six electrons.
Delving deeper into gallium's electron configuration, we find that the 3s and 3p orbitals are partially filled. The 3s orbital houses two electrons, while the 3p orbitals hold three electrons, leaving one orbital empty. This unpaired electron in the 3p orbital has profound implications for gallium's chemical properties, bestowing upon it a degree of reactivity and affinity for other atoms.
By unraveling the intricate tapestry of gallium's electron configuration, we gain invaluable insights into its chemical behavior. This knowledge serves as a cornerstone for comprehending gallium's versatile applications, ranging from semiconductors to medical imaging.
Aufbau Principle: The Energetic Hierarchy of Electrons
Just as there's a hierarchical ladder in organizations, the electrons in an atom also follow a specific order of energy levels. This order is governed by the Aufbau principle. It states that electrons prefer to occupy the lowest energy levels first before moving on to higher ones.
The Aufbau principle provides a roadmap for understanding electron configuration, the arrangement of electrons in an atom's orbitals. Starting from the innermost energy level (1s), electrons fill up the orbitals in a stepwise manner, filling the lower energy orbitals before moving to the higher energy ones.
This energy-based filling pattern ensures that electrons are as close as possible to the positively charged nucleus, resulting in a more stable atomic structure. The Aufbau principle is crucial in determining the chemical properties and behaviors of elements.
Hund's Rule: Putting a Spin on Electron Filling
In the world of electrons, there's a twist to the filling story. Hund's rule adds a layer of complexity to the Aufbau principle. It states that when electrons occupy orbitals of equal energy (such as the p orbitals), they prefer to occupy different orbitals with parallel spins.
Hund's rule plays a significant role in determining the magnetic properties of atoms. Unpaired electrons with parallel spins can lead to paramagnetism, a property where materials are attracted to magnetic fields. On the other hand, paired electrons with opposite spins result in diamagnetism, where materials are repelled by magnetic fields.
So, the Aufbau principle and Hund's rule work in tandem to paint a picture of electron configuration. They guide electrons to fill orbitals in an energy-efficient manner, ultimately shaping the chemical and magnetic characteristics of elements.
Hund's Rule: Unveiling the Secrets of Unpaired Spins
Imagine an atomic playground where tiny particles called electrons dance around a positively charged nucleus like celestial bodies in orbit. Within these intricate cosmic systems, Hund's rule emerges as an invisible choreographer, guiding the behavior and arrangement of these enigmatic subatomic particles.
Hund's rule, named after its discoverer, Friedrich Hund, is a quantum mechanical principle that dictates the spin orientation of electrons in an atom's orbitals. An orbital is an imaginary region surrounding the nucleus where an electron is likely to be found. Each orbital can accommodate a maximum of two electrons, but these electrons must have opposite spins.
Spin, analogous to the rotation of a celestial body, can be either up or down. Hund's rule states that when multiple electrons occupy the same orbital, they will prefer to have parallel spins (both up or both down). This arrangement maximizes the exchange energy, a subtle quantum effect that arises from the wave-particle nature of electrons.
The consequences of Hund's rule are far-reaching. It determines the magnetic properties of atoms. Atoms with unpaired spins, where some electrons have opposite spins, behave as tiny magnets—a phenomenon known as paramagnetism. In contrast, atoms with all spins paired are non-magnetic, or diamagnetic.
Understanding Hund's rule is essential for comprehending the electronic structure of atoms and the behavior of matter at the atomic level. It provides a glimpse into the hidden order and intricate laws that govern the submicroscopic world, influencing everything from chemical bonding to the properties of materials.
Diving into Electron Configuration: A Journey into the Building Blocks of Matter
Just as a house is built from bricks, atoms, the fundamental building blocks of matter, are constructed from even smaller particles called electrons. Understanding how these electrons are arranged is crucial for comprehending the behavior and properties of all elements. Let's embark on a storytelling adventure to unravel the mysteries of electron configuration.
The Birth of Electrons: The Atomic Family
At the heart of every atom lies a tiny, central nucleus, containing positively charged protons and neutral neutrons. Whirling around this nucleus like planets around a star are electrons, negatively charged particles with a mass that is a mere fraction of that of a proton. The number of protons an atom possesses defines its atomic number and uniquely identifies it as a particular element.
Valence Electrons: The Social Butterflies
Electrons are not confined to the nucleus. They occupy distinct regions around it, known as orbitals. The electrons in the outermost orbital, called valence electrons, play a vital role in chemical reactions. Like social butterflies, they interact with each other and electrons from other atoms, influencing the chemical behavior of the element.
Electron Configuration: A Cosmic Address Book
The arrangement of electrons in an atom's orbitals is its electron configuration. It provides a map that reveals their location and energy levels. Each orbital can hold a specific number of electrons, determined by four quantum numbers: principal, angular momentum, magnetic, and spin.
Pauli Exclusion Principle: The Invisible Barrier
Wolfgang Pauli, a brilliant physicist, formulated a fundamental principle that governs electron configuration known as the Pauli Exclusion Principle. It states that no two electrons in an atom can have the exact same set of all four quantum numbers. This principle sets a limit on the number of electrons that can occupy each orbital and ensures the stability of atomic structures.
The Periodic Table: Electron Configuration's Blueprint
The periodic table is a visual representation of all known elements, arranged according to their electron configurations. Elements in the same vertical column, known as a group, share similar electron configurations and, therefore, exhibit similar chemical properties. This allows scientists to predict the behavior of an element based on its position in the table.
Gallium: An Electron Configuration Case Study
Gallium, a silvery-white metal, has an electron configuration of [Ar]3d¹⁰4s²4p¹. This means that its valence electrons occupy the 4s and 4p orbitals, making it a reactive element. Gallium's electron configuration explains its characteristic properties, such as its high electrical conductivity and low melting point.
Electron configuration is a fundamental concept in chemistry that reveals the arrangement of electrons in atoms, providing insights into their chemical behavior and properties. Understanding the principles behind electron configuration, including the Pauli Exclusion Principle, allows us to unravel the complexities of the atomic world and its impact on the structure of all matter we encounter.
Periodic Table: A Blueprint of Elements: Describe the organization of elements and relate it to electron configuration.
Unveiling the Secrets of the Periodic Table: A Blueprint of Elements
In the realm of chemistry, the periodic table stands as a testament to the orderliness of nature. It meticulously organizes the known elements based on their fundamental characteristics, including their electron configurations.
Electron Configuration: The Keystone of Atomic Structure
Every atom is a universe in miniature, teeming with particles. The nucleus contains positively charged protons and neutral neutrons, while the surrounding cloud of electrons balances the positive charge. The arrangement of these electrons, known as their electron configuration, determines an element's chemical behavior and place in the periodic table.
The Periodic Table: A Roadmap to Element Properties
The periodic table is not merely a list of elements; it is a roadmap that reveals their relationships and properties. Elements are arranged in rows (periods) and columns (groups) based on their electron configurations. Each row represents a new energy level added to the atom.
Electrons occupy orbitals, which are regions of space where they are most likely to be found. The Aufbau principle governs the filling of these orbitals, dictating that electrons occupy the lowest energy orbitals first. This principle, along with the Pauli exclusion principle which states that no two electrons can have the same set of quantum numbers, determines the electron configuration of each element.
Gallium's Family: The Group 13 Elements
Gallium, an element with atomic number 31, belongs to Group 13 of the periodic table. This group consists of elements with three valence electrons, located in the outermost energy level. The similarities and differences in electron configurations among Group 13 elements give rise to their characteristic properties.
For instance, the valence electrons of gallium make it a good electrical conductor. Its malleability and ductility stem from the ease with which these electrons can move between atoms, allowing it to be easily shaped.
The periodic table is an indispensable tool for chemists, providing a comprehensive understanding of the structure, properties, and reactivities of elements. By deciphering the electron configurations of its occupants, we can unlock the secrets of the atomic world and harness their potential to shape our technological advancements.
Gallium's Family: Group 13 Elements: Discuss the similarities and differences in electron configurations, characteristics, and properties of gallium's group members.
Gallium's Family: Group 13 Elements
Step into the world of chemistry and let's explore the fascinating family of group 13 elements, where gallium proudly resides. These elements share a special bond, not only in their electronic configurations but also in their fascinating characteristics.
Imagine gallium as the cool kid on the block, with three unpaired electrons in its outermost shell. This unique arrangement gives gallium and its group members a charismatic flair. They're bold, standing out from the crowd with their metallic luster and silvery-white appearances.
But beyond their aesthetic appeal, these elements are far more than just pretty faces. They're versatile performers, playing vital roles in various industries. Gallium, for instance, is a star in the electronics world, gracing transistors and LEDs with its presence.
Indium, another group 13 element, deserves its own spotlight. This shiny, malleable metal is a master of disguise, mimicking the appearance of silver. In the realm of semiconductors, indium is a true rockstar, lending its talents to solar cells and thin-film displays.
Now, let's shift our focus to thallium, the elusive member of group 13. Unlike its more sociable siblings, thallium plays a more solitary role. This toxic substance demands respect and can be found in various forms, from colorless crystals to ominous-looking black crystals.
As we venture through the family tree of group 13 elements, we witness a subtle dance of similarities and differences. They embrace their three valence electrons, but their properties waltz to a unique tune. From gallium's silvery gleam to thallium's dark mystique, these elements leave an unforgettable mark on the tapestry of chemistry.
Related Topics:
- Calculating Factory Costs: Comprehensive Guide To Essential Factors And Considerations
- Mastering Solubility Rules: An Effective Guide For Memorization
- Dog Chromosome Number: Essential For Health And Genetic Inheritance
- Maximize The Longevity Of Borax Crystals: Storage Tips For Enhanced Cleaning Power
- Anatomy Of The Hard Palate: Structure, Function, And Clinical Significance