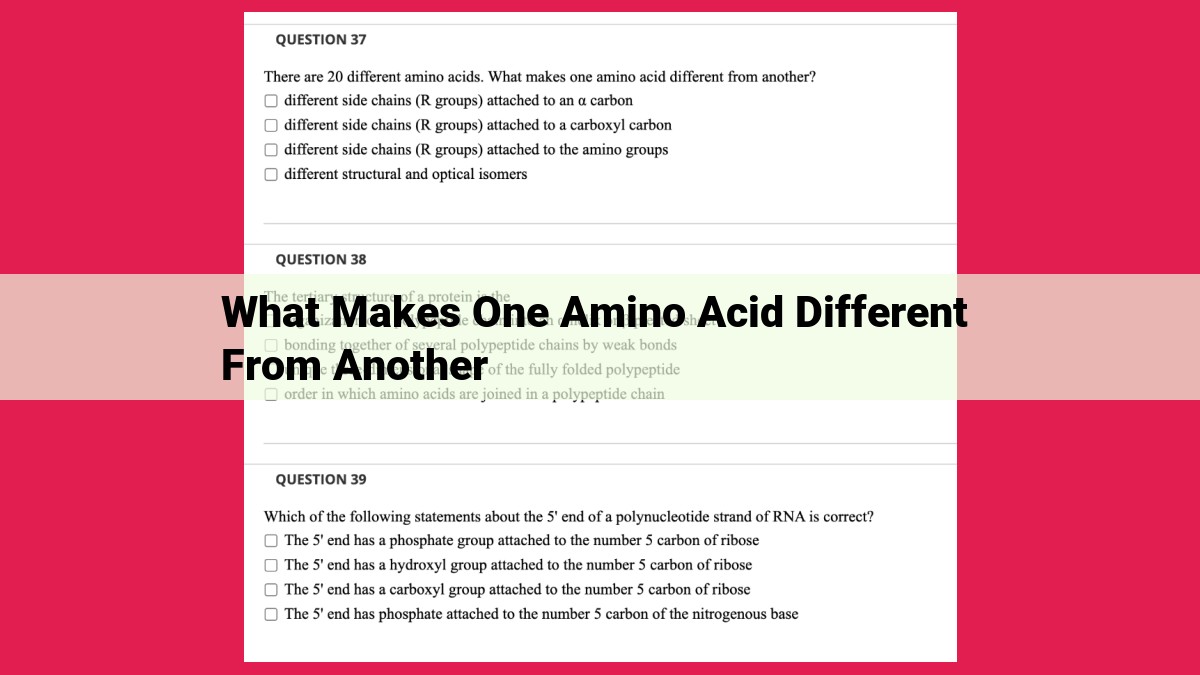
Seo-Optimized Title:understanding Amino Acids: Unraveling The Building Blocks Of Proteins
The unique nature of each amino acid stems from its distinctive side chain, which varies in composition, size, and properties. These side chains impart polarity, charge, hydrophobicity, and other characteristics that influence amino acid interactions within proteins. The size of the side chain affects protein folding and stability, while polarity determines water solubility. Hydrophobic side chains form the core of proteins, stabilizing their structure. Aromatic amino acids contribute to protein stability and interactions via their unique ring structures. Sulfur-containing side chains, particularly cysteine, enable disulfide bond formation, further enhancing protein stability. These distinct properties shape the functions and structural roles of amino acids in the complex world of proteins.
Side Chain: The Defining Feature of Amino Acids
Amino acids, the building blocks of proteins, are not just simple molecules. They possess a unique characteristic that sets them apart: side chains. These side chains are attached to the central carbon atom of each amino acid and are responsible for the vast diversity and functionality of proteins.
The side chains vary significantly in their composition, size, and properties. They can be small and simple, like the hydrogen atom found in glycine, or large and complex, like the aromatic ring found in tryptophan. The size of the side chain is an important factor in determining the overall shape and stability of a protein.
But it's not just the size that matters. The properties of the side chains also play a crucial role. They can be hydrophilic (water-loving) or hydrophobic (water-hating), negatively or positively charged, or neutral. These properties influence the interactions between amino acids and other molecules, contributing to the unique functions of each protein.
For instance, the presence of charged side chains in proteins allows them to interact with charged molecules in the environment, such as ions or other proteins. Hydrophilic side chains, on the other hand, facilitate interactions with water molecules, contributing to protein solubility and stability.
In short, the side chains of amino acids are not mere appendages. They are essential features that determine the structure, properties, and functionality of proteins. Without them, the world of biology would be a much simpler, less diverse place.
Charge: Positives, Negatives, and pH Dependence
At the heart of every amino acid lies a side chain, a fundamental feature that imparts unique properties to these building blocks of life. Among these traits, charge stands as a pivotal player in the symphony of protein structure and function.
The charge of an amino acid depends heavily on the environmental pH, a measure of acidity or alkalinity. As pH fluctuates, so too does the charge of the amino acid. This pH dependence stems from the ionization of certain side chain groups, resulting in the loss or gain of protons.
Positive charge emerges when amino acids bear positively charged side chains, such as lysine or arginine. Conversely, negative charge arises when side chains possess negatively charged groups, like glutamic acid or aspartic acid.
These charges play a crucial role in protein interactions, governing how proteins interact with each other and with other molecules. Positively charged amino acids have an affinity for negatively charged groups, while negatively charged amino acids attract positively charged molecules. This intricate dance of attraction and repulsion shapes the intricate architecture of proteins and facilitates their diverse functions.
For instance, the net charge of a protein can influence its solubility in water. Positively charged proteins tend to be water-soluble, as they form favorable interactions with water molecules. On the other hand, negatively charged proteins are often less water-soluble, as they repel water molecules.
Furthermore, charge plays a vital role in enzyme catalysis. Many enzymes rely on positively charged amino acid residues to attract negatively charged substrates, creating an environment that facilitates chemical reactions. Conversely, negatively charged amino acids can repel other negatively charged molecules, preventing them from interfering with enzyme activity.
In summary, the charge of amino acids, governed by pH, has a profound impact on protein structure and function. It influences protein interactions, solubility, and enzyme activity, highlighting the intricate interplay between the building blocks of life and their diverse roles in biological processes.
Polarity: The Key to Understanding Water-Loving and Water-Hating Amino Acids
In the realm of biochemistry, understanding the properties of amino acids is essential. Among these properties, polarity plays a crucial role in shaping the behavior of these building blocks of life.
Polarity refers to the uneven distribution of electrical charge within a molecule. Amino acids exhibit varying degrees of polarity depending on the nature of their side chains. Polar side chains have an uneven distribution of charge, creating a partial positive or negative charge. On the other hand, nonpolar side chains have a relatively uniform distribution of charge, resulting in no net charge.
The polarity of amino acids profoundly influences their interactions with other molecules. Polar amino acids are hydrophilic, meaning they attract water molecules. This property is essential for the solubility of proteins in water. Proteins with a high content of polar amino acids are more likely to be soluble and interact with water-based environments, such as the cytoplasm of cells.
In contrast, nonpolar amino acids are hydrophobic, meaning they repel water molecules. They prefer to interact with other nonpolar molecules, such as lipids and hydrocarbon chains. Hydrophobic amino acids are often found in the interior of proteins, forming a hydrophobic core that stabilizes the protein structure and prevents it from dissolving in water.
Understanding the polarity of amino acids is fundamental for comprehending the structure and function of proteins. Polar amino acids allow proteins to interact with water-based environments, while nonpolar amino acids play a crucial role in maintaining protein stability and preventing aggregation. By appreciating the concept of polarity, we gain a deeper understanding of the intricate workings of life's molecular machinery.
Size: A Balancing Act for Protein Structure
The size of amino acid side chains plays a crucial role in determining a protein's structure, stability, and flexibility.
Impact on Protein Folding:
The size and shape of side chains influence how proteins fold. Smaller side chains allow for closer packing, facilitating the formation of alpha-helices and beta-sheets. In contrast, larger side chains create steric hindrance, preventing close packing and promoting more open structures.
Stability and Flexibility:
Protein stability relies on a delicate balance of forces. Larger side chains contribute to increased stability by forming more hydrophobic interactions, which are favored in the interior of proteins. However, very large side chains can disrupt the protein's hydrophobic core, weakening stability.
Conversely, smaller side chains enhance flexibility by reducing steric clashes. This allows for greater conformational changes, crucial for protein functions such as binding and catalysis.
Balancing Act:
Protein structure is thus a balancing act between stability and flexibility. The judicious distribution of side chains of varying sizes ensures that proteins maintain the optimal balance between these opposing forces. Small side chains provide flexibility, while large side chains contribute stability, creating a dynamic equilibrium that sustains protein function.
Hydrophobicity: The Key to Protein Cores
In the intricate realm of proteins, there exists a hidden compartment—the hydrophobic core. Encapsulated within this core is a group of amino acids with a remarkable property: hydrophobicity. These hydrophobic amino acids, like shy and reserved guests at a grand ball, prefer to avoid contact with water, the ubiquitous solvent of life.
Their aversion to water stems from the nonpolar nature of their side chains. Unlike polar side chains, which harbor charged or uncharged hydrophilic groups, nonpolar side chains are uncharged and devoid of functional groups that interact favorably with water. Therefore, in an aqueous environment, these nonpolar side chains huddle together, shunning water like oil repels vinegar.
This hydrophobic clustering serves a vital purpose in protein structure. By sequestering nonpolar side chains within the protein's core, the protein becomes more stable and resistant to denaturation. The hydrophobic core acts as a protective force field, shielding the protein's delicate interior from the disruptive effects of water.
Additionally, the hydrophobic core plays a crucial role in protein folding. As a protein's constituent amino acids seek their lowest energy state, the nonpolar side chains drive the folding process by minimizing their contact with water. This hydrophobic collapse brings together the protein's distant regions, eventually leading to its characteristic three-dimensional structure.
In summary, hydrophobicity is a key property of amino acids that orchestrates protein structure and stability. By forming a hydrophobic core, these nonpolar side chains shield the protein's interior from water and facilitate its efficient folding, ultimately enabling proteins to perform their diverse biological functions.
Aromaticity: Special Structures with Unique Roles
In the realm of amino acids, there exists a captivating class known as aromatic amino acids. These enigmatic molecules possess a unique structural feature that sets them apart from their counterparts. Their defining characteristic lies within their aromatic rings, which confer stability and bestow upon them a pivotal role in the intricate world of protein interactions.
The aromatic amino acids present in the genetic code number three: Tryptophan, Tyrosine, and Phenylalanine. Their aromatic rings are composed of alternating double and single bonds, creating a resonance structure that endows them with extraordinary stability. This stability grants them the ability to withstand environmental fluctuations and maintain their structural integrity amid the bustling cellular milieu.
One of the most intriguing properties of aromatic amino acids is their involvement in protein interactions. Their aromatic rings are like molecular magnets, capable of engaging in a specialized interaction known as pi-stacking. Picture these amino acids as Lego blocks that specifically interlock with one another through their aromatic surfaces. This interaction plays a crucial role in stabilizing protein structures and dictating their overall architecture.
For instance, in the hydrophobic core of proteins, aromatic amino acids form a tightly packed network through pi-stacking. This aromatic core shields delicate parts of the protein from the watery environment outside and contributes to the overall stability of the protein. Moreover, these aromatic interactions are often crucial for the formation of binding pockets and enzyme active sites, allowing proteins to interact with other molecules and perform their biological functions.
In summary, the aromatic amino acids are a fascinating class of molecules that play a pivotal role in protein structure and function. Their unique aromatic rings provide stability and facilitate protein interactions through pi-stacking, shaping the intricate tapestry of life at the molecular level.
Sulfur Content: A Keystone for Protein Stability
Amino acids, the building blocks of proteins, possess varying properties that contribute to their unique roles in biological systems. Among these properties, sulfur content plays a pivotal role in the stability and function of proteins.
Sulfur-containing amino acids, primarily cysteine and methionine, stand out due to their ability to form disulfide bonds. Disulfide bonds are covalent linkages between two sulfur atoms, creating strong and stable connections within proteins. These bonds play a crucial role in maintaining protein structure and mediating protein-protein interactions.
In the protein folding process, disulfide bonds stabilize the tertiary and quaternary structures by locking specific regions of the polypeptide chain into place. This structural stability enhances the protein's resistance to denaturation and proteolytic degradation. Additionally, disulfide bonds are essential for the proper function of many proteins, such as antibodies, hormones, and enzymes, where they facilitate correct protein conformation and ligand binding.
Furthermore, cysteine residues are central to cellular redox reactions. Cysteine's ability to undergo reversible oxidation, forming oxidized and reduced forms, enables proteins to participate in cellular processes such as DNA repair, signal transduction, and regulation of gene expression.
In summary, sulfur-containing amino acids and the disulfide bonds they form are indispensable for protein stability and functionality. Their unique properties contribute to the maintenance of protein structure, facilitate protein interactions, and enable redox reactions essential for cellular processes.
Related Topics:
- Pronouncing Medical Terms Correctly: A Comprehensive Guide To Dupuytren’s Contracture
- The Significance And Interpretation Of “Sx” In Statistics: A Guide To Sample Mean And Related Concepts
- Eukaryotic Cell Cycle: Mitosis, Cytokinesis, And Genetic Stability
- Decigram To Gram Conversion: A Comprehensive Guide For Accurate Measurement
- Pronunciation Guide For Gastrocnemius: Understanding The Calf Muscle Anatomy